Lithium-ion batteries (LIBs) have become the cornerstone of modern portable energy due to their compact size, high energy density, rechargeability, and cost-effectiveness. Their adoption has skyrocketed in recent years, and the United States is a top global consumer, importing over 235,000 metric tons of LIBs in the last quarter of 2023 alone [1]. Demand is dominated by the electric vehicle (EV) industry, but LIBs also power a wide array of everyday devices, from smartphones and laptops to stationary battery energy storage systems, such as Powerwalls. The exponential rise in demand for these batteries is driven by the global shift toward cleaner energy sources and the proliferation of consumer electronics and micro-mobility devices (e.g., electronic bikes, scooters, wheelchairs, and skateboards). As technology advances, these batteries further cement their role in our daily lives and the future of sustainable energy.
The prospect of building a significantly more sustainable and potentially renewable power grid via widespread electrification is a noble endeavor. An often-overlooked aspect of this relatively new technology, however, is what happens when this equipment fails. Whether it be from end-of-life degradation, damaged/defective/recalled (DDR) cells, or physical or thermal insult, all LIB cells have a shelf life that largely depends on build quality. As it stands, over 80% of US-imported LIBs come from China, where industry regulations and quality control standards are often lacking [2]. Technologically advanced batteries and chargers will include built-in battery management systems that can regulate power supply and prevent overcharging as well as over-discharging, two methods of electrical abuse that can cause instability within the cell and lead to short-circuiting. Quality checks and safety components, however, are often much more expensive to produce at scale and, at this point, are not a requirement in most consumer electronics. This means that most LIB devices on a 50% Chinese-driven market like Amazon—items such as flashlights, power tools, and electric scooters—have an inherently greater risk of failure compared to more safely made products [3].
In addition to the potential for electrical abuse, LIBs are prone to mechanical, thermal, and moisture-related insults, all of which lead to short-circuiting. A mechanical insult occurs when a cell is crushed or pierced, and a thermal insult can arise if a cell is exposed to just 140 degrees Fahrenheit and above—a temperature likely to be reached inside shipping containers before a cell is even used [4]. When any of these short-circuit scenarios occur, a great amount of heat is generated within the cell. This heat can cause the cell’s internal components to degrade and then react with each other, generating a large amount of the flammable byproduct hydrogen and exponentially more heat. This exothermic reaction can quickly reach hydrogen’s autoignition point, resulting in a self-sustaining fire that can burn in the absence of external oxygen (i.e., underwater) while emitting enormous amounts of toxic gases in a process deemed “thermal runaway.” When a reactive cell insults its neighboring cells, explosions and domino-like thermal runaway propagation can occur.
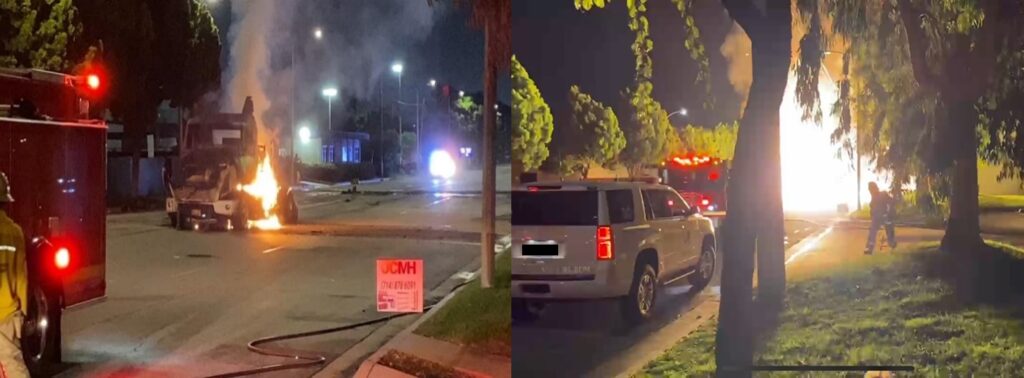
Understanding Lithium-Ion Battery Fires
The violent exothermic reactions that occur in these chemically driven processes are just one part of the story, and first responders are working diligently to understand the risks of exposure in the field. In a two-day interagency field experiment hosted by the San Diego Fire Department, US Environmental Protection Agency (EPA) Region 9 and their Superfund Technical Assessment & Response Team (START) contractor, Weston Solutions, Inc. (Weston), recorded a host of disturbing air monitoring and sampling results that shed light on just how toxic these battery fires can be.
The field experiment was conducted on the San Diego Sheriff’s firing range and consisted of manually instigating thermal runaway in various sizes and arrays of LIBs within an 8’x8’x8′ burn chamber. Additional variables tested include state of charge (SOC), common battery chemistries (lithium iron phosphate versus nickel manganese cobalt), and manufacturer.
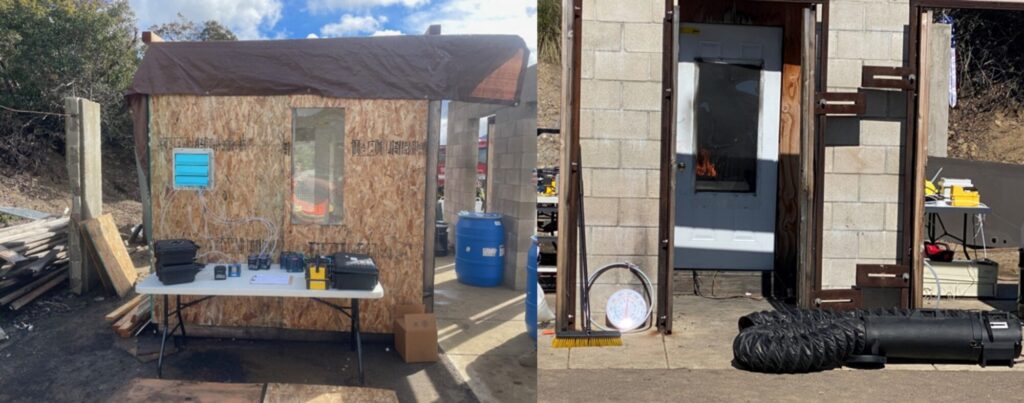
Hydrogen fluoride (HF) gas is a toxic and corrosive byproduct of LIB fires. It can cause severe health issues, such as irregular heartbeats and fluid buildup in the lungs, which can be fatal. The Occupational Safety and Health Administration (OSHA) allows a maximum exposure limit of 3 parts per million (ppm) for HF, while the Centers for Disease Control and Prevention (CDC) National Institute for Occupational Safety and Health (NIOSH) considers 30 ppm to be “immediately dangerous to life and health” (IDLH)[5].
The field study found air monitoring results for HF that maxed out the sensors at 20 ppm, with sampling results of up to 43 ppm HF during various LIB burn tests. In real-world emergency responses, there have now been several instances where the public was advised to either shelter in place or evacuate due to the risk of HF vapor clouds migrating from LIB fires. Additionally, the field study found air sampling results for metals where cobalt and nickel approached or exceeded IDLH conditions.
The insights gleaned from this field study are now being used to create accurate plume models for community safety and have received recognition from dozens of governmental agencies and industry partners, including CDC-NIOSH, which is planning on conducting similar research aimed largely at first responder safety for EV fires. Through these studies, CDC-NIOSH hopes to eventually propose regulatory health and safety standards specific to LIB fires [6].
When it comes to LIBs, the incredible heat generated, the toxic byproducts, and the self-sustaining nature of thermal runaway fires are what make them uniquely difficult to manage. Additionally, once a battery pack—recognized as more than a single cell in series or parallel powering a device or devices—is compromised by thermal, mechanical, or electrical means, there is no way of telling exactly when the cells may undergo thermal runaway or explode. They become a sort of “ticking time-bomb,” and reports of battery fires occurring days, weeks, months, and even a year after the device’s initial insult are all too common. This combination of toxicity, unpredictability, and imperviousness to water has led most fire departments to adopt a “stand back and let it burn” approach with LIB fires. There are cases where the cooling effect of water may be a tool in preventing the propagation of the fire to additional cells and/or neighboring valuables, but there is currently no known method or substance that is effective at extinguishing an LIB fire.
Post-Fire Remediation Strategies – The Maui Method
Since early 2023, EPA Region 9 and Weston (START contractor) have been involved in the remediation of over a dozen LIB sites. From EV fires to electric scooter fires, and even battery cleanups resulting from the 2023 Maui, HI Wildfires, the remediation process for LIBs has evolved and expanded to a complex decision tree that balances safety, practicality, and cost-effectiveness.
For example, EPA Region 9 and Weston addressed the risk involved in shipping DOT-approved containers housing DDR batteries after the 2023 Maui Wildfires. Most processes involve packaging DDR batteries in special Department of Transportation (DOT)-approved containers for transport to waste facilities or recyclers. At recycling facilities, these batteries are then de-energized and dismantled to recover about 95% of their precious metals. However, these DOT-approved containers and shipping methods are not always safe. DDR batteries can still produce flammable hydrogen even after being burned or destroyed. This is risky when transporting large quantities, as hydrogen can fill a container and explode with the smallest of sparks. Therefore, DOT-approved methods for shipping DDR batteries still pose a significant risk.
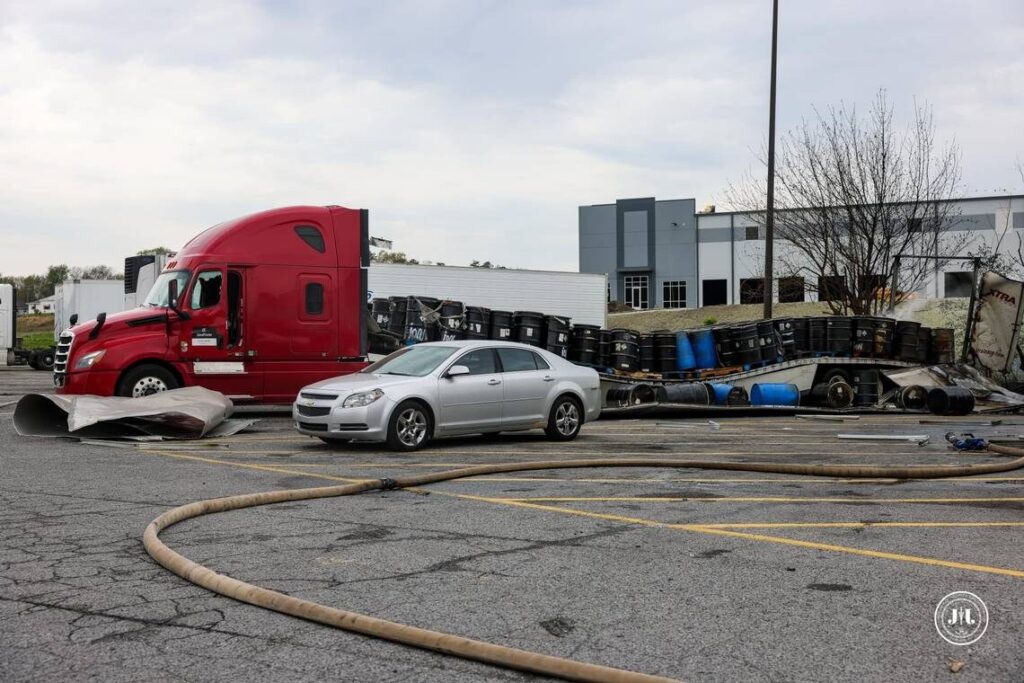
Following the 2023 Maui Wildfires, the Federal Emergency Management Agency (FEMA) assigned EPA to handle hazardous material removal, including LIBs. Due to the lack of facilities in Hawaii, the hazardous materials had to be shipped to the mainland for proper disposal. In the case of batteries, however, maritime shippers refused to accept even properly labeled and packaged DDR LIBs for fear of a thermal runaway event while at sea. Aware of the threats surrounding thermal runaway in DDRs during transport, EPA and Weston developed a way to render 30 tons of LIBs into a safer-to-ship material that was acceptable to shippers, DOT, and LIB recyclers. They did this by bringing the first two steps of an LIB recycling facility, brining and dismantling, directly to the field. This first-of-its-kind approach to remediating an LIB site is now coined “The Maui Method.”
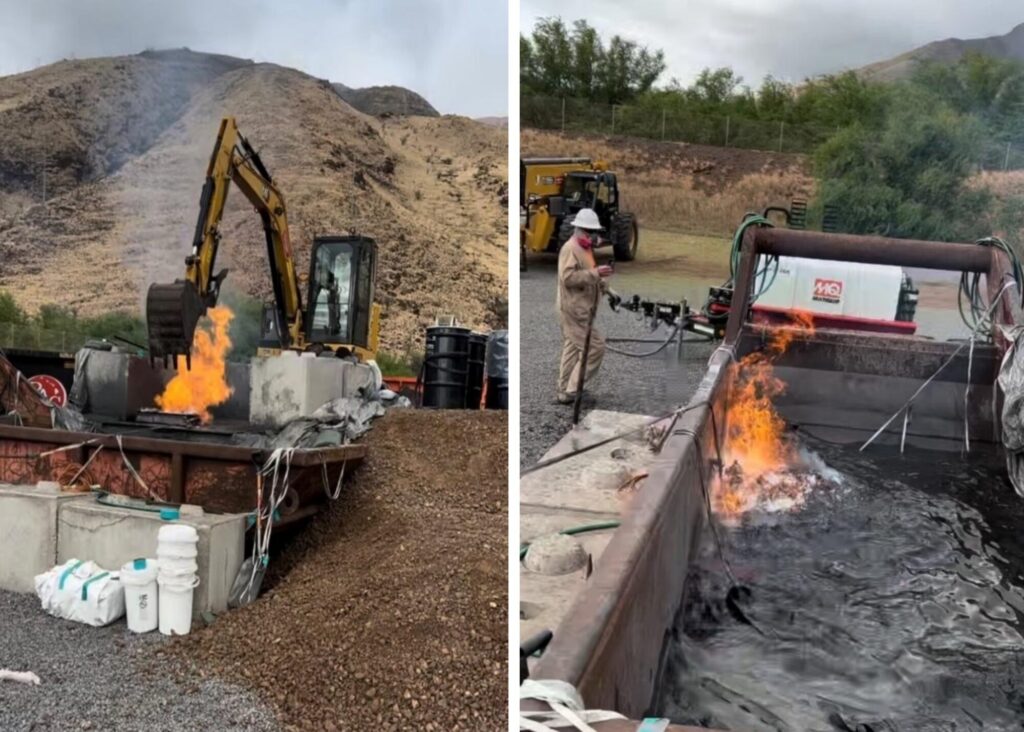
Field brining/de-energizing is a fairly simple process and is necessary to bring down a cell’s SOC before dismantling it. Research shows that LIBs with a lower SOC tend to have a lower heat release rate when undergoing thermal runaway, which helps to prevent neighboring cell propagation [6]. Because most LIB recycling facilities have a protected proprietary brine solution, EPA and Weston experimented with different brine solutions and concentrations until one was found to consistently lower a battery’s SOC from approximately 4.0 volts (V) to 1.0 V in about three days. In Maui, roughly 500 gallons of solution effectively reduced SOC for all 30 tons of LIB material without ever needing to be recharged or swapped out.
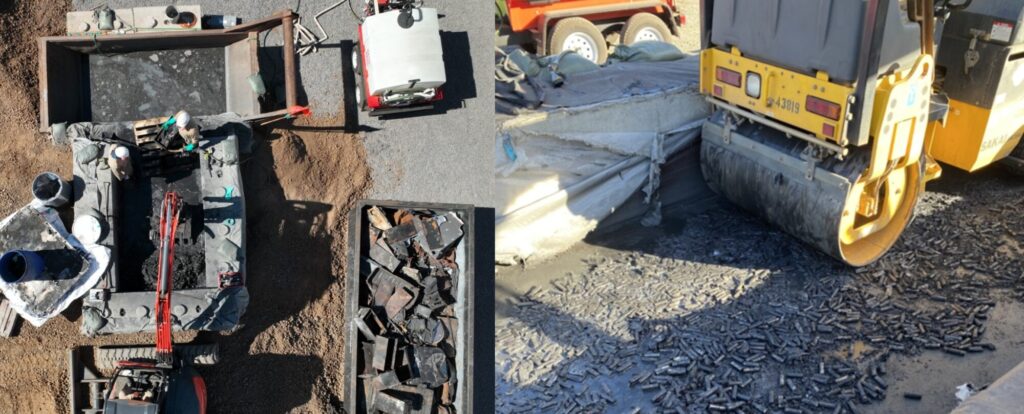
Field dismantling, or crushing, was straightforward. Using the Maui Method, batteries were identified, extracted, and safely transported to a dedicated staging area. They were submerged in brine for up to three days, then crushed with a steam roller until they no longer held a charge or met DOT/EPA battery definitions. Scrap battery materials were collected in ventilated drums or boxes to allow hydrogen to escape while keeping moisture out. The final product was placed in open-top containers for complete hydrogen venting. This DOT-approved process ensured no thermal events, and the LIB recycler accepted the scrap. All 30 tons of LIB material from the Maui Wildfires—including 94 EVs and 274 Powerwall batteries—were processed this way, and the leftover brine solution did not exceed regulatory limits for hazardous waste.
Watt to Know Next?
Knowing that LIBs can be dangerous and unpredictable is the first step toward hazard mitigation. For their overwhelming prevalence in our everyday lives, the number of incidents that do occur is relatively small, but they now receive an intense amount of media scrutiny that has prompted innovative and proactive regulations for personal and commercial use alike.
San Francisco, CA, now requires all powered mobility devices (PMDs), such as electric scooters, to have safety-certified batteries. Additionally, new San Francisco fire codes state that no more than four PMDs can be charged inside a single dwelling unit at a time without additional fire safety systems (e.g., sprinklers) and that PMDs must be plugged directly into the wall (not into power strips or extension cords); must use original batteries and chargers, not recalled or refurbished batteries; and cannot be disposed of in landfill, recycling, or compost bins but must be taken to an LIB recycler [7].
As consumers, awareness of these potential hazards will hopefully lead to smarter purchases, opting for products that use batteries from safety-oriented manufacturers instead of whatever is cheapest. Big-name manufacturers like Samsung are typically a safer bet, whose batteries and devices are now produced with rigorous quality control standards after their 2016 exploding Galaxy Note 7 smartphone issue. Additionally, the US House of Representatives recently passed a bill that is forcing the Consumer Product Safety Commission to establish consumer safety standards for LIBs within the next year [8]. Until those standards are fully established and enforced, the majority of LIBs on the market will remain unregulated, and it is up to local governments and consumers to encourage safe purchase, storage, charging, and disposal practices for our own safety.
Collaboration between manufacturers, regulatory bodies, and emergency services is crucial to continuously updating safety standards and response strategies as this technology evolves, and responsibility also falls on the consumer to follow these rules and recommendations for our own good. By taking these steps, the risks associated with LIBs can be mitigated, and the safety of communities enhanced.
References
- China continues to dominate US lithium-ion battery imports as tariff hike looms | S&P Global Market Intelligence (spglobal.com)
- U.S.: lithium-ion battery imports by country 2024 | Statista
- Amazon’s “Significant” Reliance on Chinese Sellers – Marketplace Pulse
- How Hot Do Shipping Containers Get? – TheShip.ai
- CDC – NIOSH Pocket Guide to Chemical Hazards – Hydrogen fluoride
- National Firefighter Registry (NFR) for Cancer Quarterly Newsletter (Summer 2024) | NIOSH | CDC
- Lithium-ion Battery Safety | SF Fire Website (sf-fire.org)
- Text – H.R.1797 – 118th Congress (2023-2024): Setting Consumer Standards for Lithium-Ion Batteries Act | Congress.gov | Library of Congress